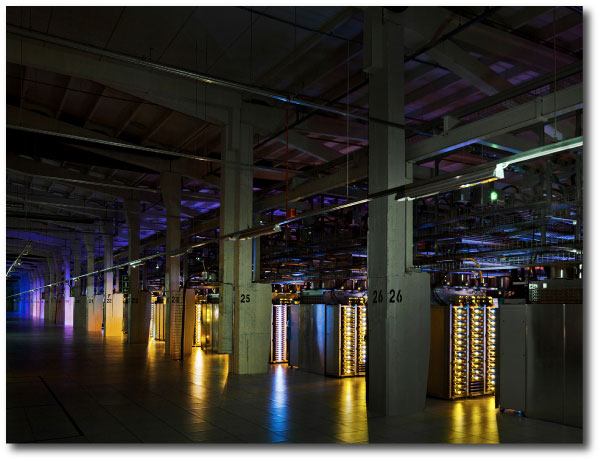
Above: A Google Data Center Image source.
A few weeks ago, there was an interesting article in the New York Times.
On the flat lava plain of Reykjanesbaer, Iceland, near the Arctic Circle, you can find the mines of Bitcoin.
To get there, you pass through a fortified gate and enter a featureless yellow building. After checking in with a guard behind bulletproof glass, you face four more security checkpoints, including a so-called man trap that allows passage only after the door behind you has shut. This brings you to the center of the operation, a fluorescent-lit room with more than 100 whirring silver computers, each in a locked cabinet and each cooled by blasts of Arctic air shot up from vents in the floor.
The large-scale Bitcoin mining operation described in the article gravitated to Iceland in part because of the cheap hydroelectric power (along with natural air conditioning, the exotic-location marketing style points, and a favorable regulatory environment). Bitcoin mining is part of a emergent global trend in which the physical features and the resource distribution of the planet are being altered by infrastructure devoted to the computation that occurs in data centers. As an example, here is a map showing new 6, 11, and 18 GHz site-based FCC microwave-link license applications during the past three years.

The Western terminus of the triangle is a mysterious building (read data center) just a mile or so south of Fermilab (for more information see this soon-to-be-published paper of mine co-authored with Anthony Aguirre and Joe Grundfest).
Data Centers are currently responsible for about 2% of the world’s 20,000 TWH yearly electricity consumption, which amounts to roughly 1.4×10^24 ergs per year. If we use the Tianhe 2 computer (currently top of the list at top500.org, with a computational throughput of 33.8 petaflops, and a power usage of 17,808 kW) as a forward-looking benchmark, and if we assume that a floating-point operation consists of ~100 bit operations, the data centers of the world are carrying out 3×10^29 bit operations per year (70 moles per second).
I’ll define a new cgs unit:
1 oklo = 1 artificial bit operation per gram of system mass per second
Earth, as a result of its data centers, is currently generating somewhat more than a microoklo, and if we take into account all of the personal devices and computers, the planetary figure is likely at least several times that.
I think it’s likely that for a given system, astronomically observable consequences might begin to manifest themselves at ~1 oklo. The solar system as a whole is currently running at ~10 picooklos. From Alpha Centauri, the Sun is currently just the nearest G2V star, but if one strains one’s radio ears, one can almost hear the microwave transmissions.
Landauer’s principle posits the minimum possible energy, E=kTln2, required to carry out a bit operation. The Tianha-2 computer is a factor of a billion less efficient than the Landauer limit, and so it’s clear that the current energy efficiency of data centers can be improved. Nevertheless, even if running near the Landauer limit, the amount of computation done on Earth would need to increase several hundredfold for the Solar System to run at one oklo.
So where to look? Three ideas come to mind in increasingly far-out order.
(1) Dyson spheres are the perennial favorite. Several years ago, when the WISE data came out, I worked with two high-school students from UCSC’s Summer Internship Program to search the then newly-released WISE database for room-temperature blackbodies. To our surprise, it turns out that the galactic disk is teeming with objects that answer to this description:

(Some further work revealed that they are heavily dust-obscured AGB stars.)
(2) Wait long enough, and your data center will suffer an impact by a comet or an asteroid, and computational hardware debris will begin to diffuse through the galaxy. In the event that this happened regularly, then it might be possible to find some interesting microscopic things in carbonaceous chondrites.
(3) The T in Landauer’s principle suggests that cold locations are better suited for large-scale computation. Given that here on Earth a lot of cycles are devoted to financial computation, it might also be relevant to note that you get a higher rate of return on your money if your bank is in flat space time and you are living in a region of highly curved spacetime.