
Image Source.
Most hot Jupiters have orbital eccentricities near zero because the tidal forces exerted on them by their parent stars are strong enough to rapidly circularize their orbits. Any planet whose orbit has been circularized should also be spin-synchronous, and so like our Moon with respect to the Earth, it should turn on its axis once every trip around the star. Synchronicity lends each hot Jupiter a permanent day and night side. This likely imparts a profound effect on both the planetary weather, and on the brightness of the planet when viewed in the infrared at different orbital phases.
All of the planets observed so far with the Spitzer Space telescope have nearly circular orbits, and hence are in (or are very near) the spin-synchronous state. We’re waiting to hear the results of our Spitzer GO-4 application to observe the highly eccentric planet HD 80606b, during an upcoming ‘606 day. If our observing proposal gets a thumbs-up, it’ll dramatically broaden the range of conditions under which planets have been observed. Very shortly, I’ll be posting the results of calculations that Jonathan Langton and I have been doing which predict what the light curve of HD 80606 should look like during the periastron passage in the various Spitzer bands. Here’s a sneak preview of how the temperature distribution on the planet might evolve over a 36-hour period as seen from a direction consistent with our line of sight from the Earth:
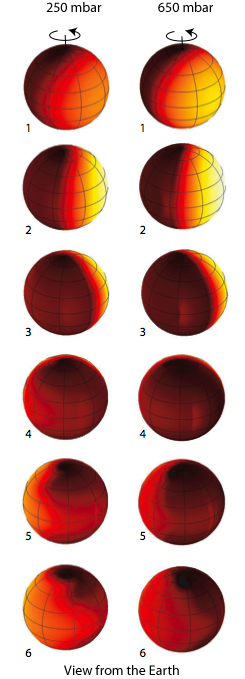
In looking over the latest officially published additions to the catalog of extrasolar planets, I noticed that there’s a very interesting object — HD 118203b — that straddles the extremes of the circular hot Jupiters and the ultra-eccentric HD 80606b. This planet was discovered in 2005 by the Swiss Team, has an orbital period of 6.13 days, a mass at least twice that of Jupiter, and a well-determined eccentricity, e=0.3. HD 118203b therefore won’t be spin-synchronous. Rather, as is also the case with HD 80606b (see the diagram here), it’ll have been forced into a state of pseudo-synchronous rotation, in which it does its best to keep one face toward the star during the periastron passage. Its day should be 64.8% as long as its year:
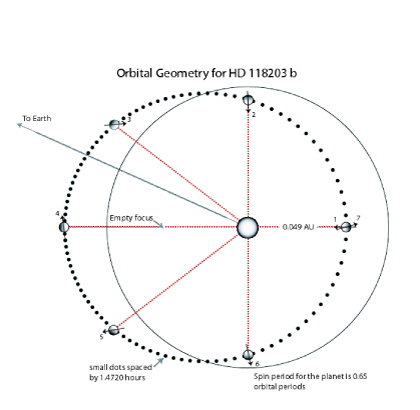
Higher resolution .eps version here.
Which raises a rather interesting question: Why is HD 118203’s eccentricity so high?
Assuming that the planet has a similar structure to Jupiter, the equations of tidal dissipation (see here for a discussion) indicate that the planet’s orbit should circularize in a mere 10-20 million years. This time scale is surprisingly short because the parent star is a subgiant with a radius ~1.5 times larger than the Sun. Something must be exerting a very strong perturbation to keep this planet’s e up.
In their discovery paper, Da Silva et al remark that the residuals around the best 1-planet Keplerian fit to the data are very large. It’s quite straightforward to verify this with the downloadable Systemic console (try it!) Da Silva and company were able to improve their fit by including a linear drift of 49.7 meters per second with their one-planet model. This corresponds to adding the effect of an outer planet that has been observed for only a small part of a single orbit. (The 43 published velocities span a period of 1.1 years.) They speculate that an outer as-yet-uncharacterized planet provides the gravitational perturbation that maintains the high eccentricity for the inner planet.
Last year, Fred Adams and I wrote a computer code (see these papers 1, 2) that includes the effect of general relativistic corrections on long-term planet-planet gravitational interactions. It’s easy to use this program to calculate what the long-term influences of various companion planets would have on HD 118203 b’s eccentricity. I ran a few trial cases, and quickly found that the interactions produced by companions that also provide the observed linear drift in the radial velocities don’t seem to be strong enough to explain HD 118203b’s high eccentricity. Could there be another explanation?
This is the sort of situation where the collaborative systemic back-end is extremely useful. I had a look at the stable fits that have been submitted so far for HD 118203. The best stable, self-consistent fit was uploaded back in October by the user Flanker, and has a reduced chi-square statistic of 1.96:
.
This fit might point in an interesting direction for some further inquiry. Instead of using a linear trend to soak up the residuals to the one-planet fit, Flanker added two additional planets. One of the planets has a mass of 0.3 Jupiter masses and is orbiting with a period of 15 days. Its periapse is nearly aligned with the periapse of the inner planet. The resulting short-period secular interaction may well be strong enough to keep the eccentricity of the innermost planet high in the face of tidal dissipation. Flanker’s model also contains an outer planet with an orbital period of 244 days and a minimum mass 0.6 times that of Jupiter.
I think it’s worthwhile to explore additional models for this system that contain planets with short enough periods to intereact strongly with the 6.13 day planet. If the perturbing body has a relatively short-period orbit, then its presence will not be hard to verify with additional radial velocity observations of the star. And also, if Spitzer’s cryogen holds out, HD 118203b might be a very interesting target for a full-phase campaign.