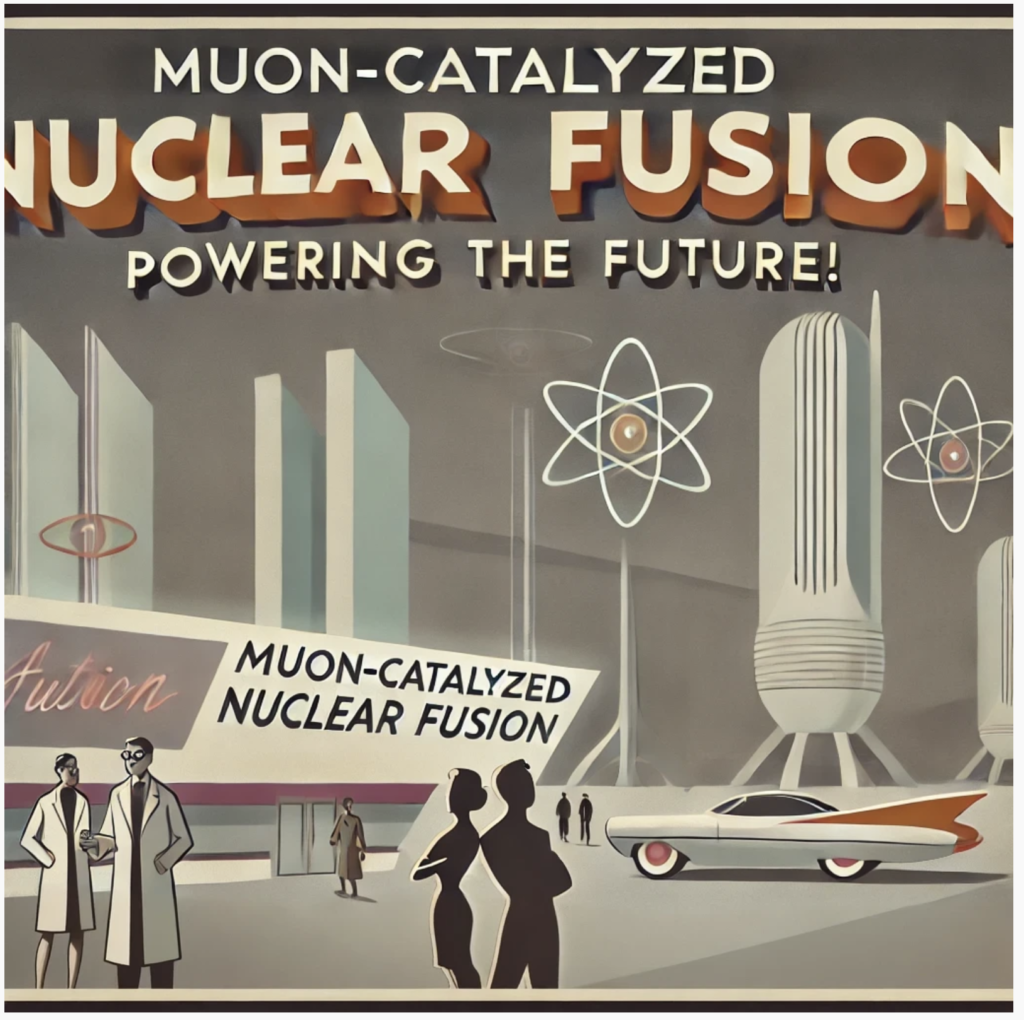
So close yet so far away.
Cold fusion of that late-80s Fleischmann-Pons electrochemical variety has long since been given over to cranks, but the term itself dates to a 1956 New York Times article describing the then-newly discovered phenomenon of muon-catalyzed fusion.
Oklo readers will recall that the muon is a negatively charged lepton with mass 105.66 MeV/c² (~200x that of the electron). On average, a muon lasts 2.2 microseconds before decaying into an electron and a neutrino via the weak interaction.
During its ephemeral lifespan, a muon can replace one of the electrons in a hydrogen molecule (generally of the exotic deuterium-tritium variety) allowing the two nuclei to draw far closer than the normal covalent bond would allow. With proximity thus achieved, the probability of deuterium-tritium fusion is greatly increased. After a fusion reaction occurs, the responsible muon is free to catalyze further events until it either decays or is removed from the action by “sticking” to an alpha particle produced by the fusion. Economic viability of the process for creating energy would require that a single muon catalyze hundreds of fusion events before it decays, a rate of efficiency that exceeds best efforts by at least a factor of two or three.
Muon-catalyzed fusion was originally observed in laboratory experiments and described in this article by Luis Alvarez et al., and was studied in depth by John David Jackson, he of Jackson’s Electrodynamics fame. Jackson’s 1957 Physical Review article is a standard reference, and remarkably, more than half a century later, he summarized the history of the field in this 2010 review.
The prospects for muon-catalyzed fusion as an energy source seemed moderately bright during the 1980s and 1990s, following the elucidation and observation of molecular states of the deuterium-tritium-muon positive ion. But then the field seemed to stall out around the turn of the millennium, as workable schemes for either producing the muons more cheaply, or improving their catalytic efficiency failed to emerge.
At the close of the 2010 article, however, Jackson sounded optimistic, writing, “The effort for such a specialized field has been prodigious, especially in the last 30 years. On the applied side, ideas continue on how to increase the number of fusions per muon and design hybrid systems to get into the realm of net energy production.”
Given all that talk these days surrounding training and inference costs, it’s fair to state that development of a scheme to get into that realm of net energy production would be quite an unexpected something.
Back in 2017, we were trying to keep the Metaculus website afloat, and we were scrambling to write questions faster than they were being resolved. For an all-best-intentions span of about a week or so, I stuck to a regime of producing a question per day, while simultaneously trying to keep them high-tone and novel. On August 28th, I managed to keep that streak going with a question on muon-catalyzed fusion, asking:
Prior to Jan 1, 2020, will a peer-reviewed article appear in the mainstream physics literature which discusses a discovery of a physical phenomenon or which outlines an engineering technique that can either (1) increase the number of deuterium-tritium fusions per muon, or (2) decrease the energy cost of muon production to the point where a break-even reactor is feasible?
Soon enough, the resolution date was imminent, and so Anthony had a look at the literature, which prompted a prompt lapse of reply on my part followed by a very belated assessment:
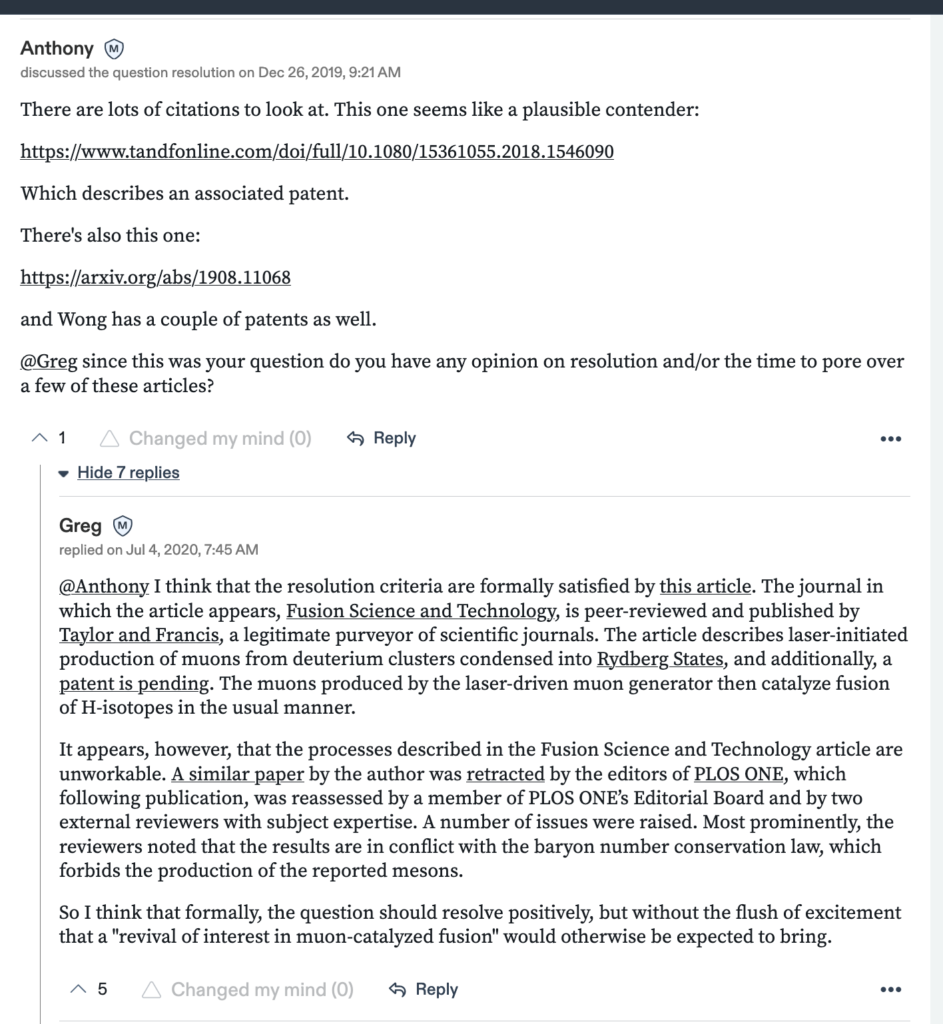
We decided to resolve the question positively, using what was essentially lawyerly splitting-hairs logic that proved very unpopular with the Metaculus crowd. I think that was the end of my career as a question-resolver.
The muons periodically come to mind, however, which prompts me to have a cursory look to see whether anything has happened. This morning, I came across this 2021 J. Phys. Energy article by Kelly, Hart and Rose, which gives a practical assessment of the (relatively) up-to-date prospects for getting muon-catalyzed fusion to work. They point to an important engineering hack that can improve efficiency — one surrounds the reaction chamber with a lead-lithium blanket that absorbs the neutrons from the fusion reactions to induce the formation of tritium, helium and heat via:
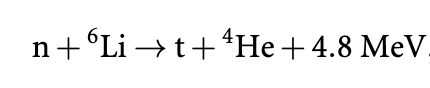
The extra heat boosts the energy output per fusion reaction from 17.6 MeV to 26 MeV. After working through various practicalities, they close with a simple daunting graph:
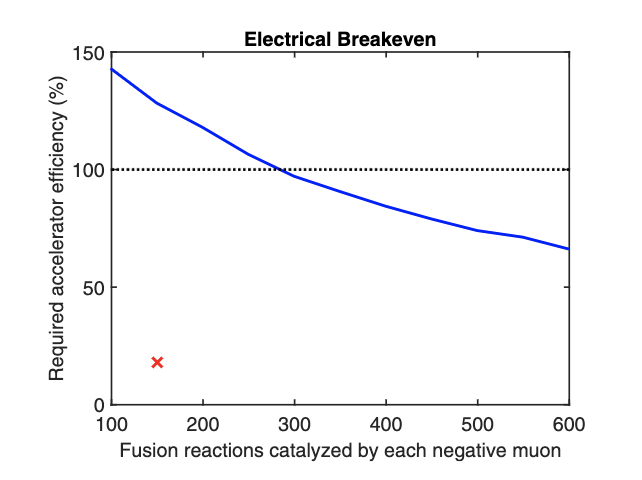
The red x marks the spot where things currently stand. If that red x can somehow be pushed up above the blue line, then muon-catalyzed fusion is a go…